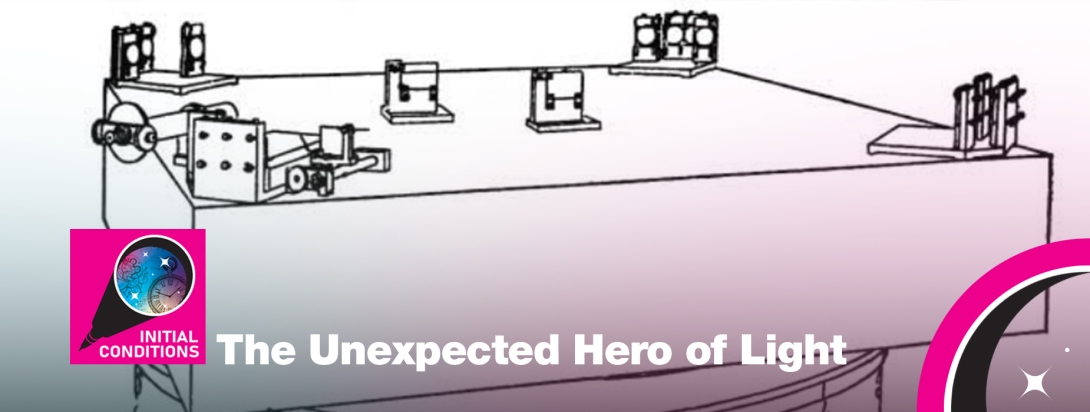
This is the story of how a Pittsburgh steel worker became the lensmaker behind some of the most important experiments of 19th century physics. John Brashear fell in love with the night sky as a kid in the 1840s. Though he took a job as a millwright, in his free time, he and his wife dedicated themselves to making a telescope lens so they could view the stars. With only an elementary education (and the mentorship of Samuel Langley at the Allegheny Observatory), John became one of the world’s preeminent opticians. His lenses were commissioned for telescopes, spectrographs, and, most significantly, for the famous Michelson-Morley Experiment which failed to detect the luminiferous aether. The dismissal of the aether may have set up Albert Einstein’s theory of relativity and modern physics. Not only was John Brashear a talented lensmaker, he was also dedicated to making the stars and the night sky accessible to all. He gave free astronomy lectures, visited schools and churches, and even a prison to ensure that regardless of someone’s situation, they could appreciate the beauty of the stars. Through his optical devices and outreach, John Brashear facilitated better access to celestial objects. This episode also features a tour of the observatory John helped build, the Allegheny Observatory, led by Lou Coban.
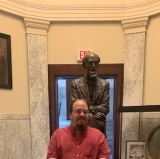
Lou Coban is the manager of the Allegheny Observatory and has been working at the Observatory maintaining telescopes, assisting research and courses, and providing public tours and lectures for almost 30 years.
“Initial Conditions” Episode 9, Allegheny Observatory By: Maura Shapiro and Justin Shapiro
Date: August 30, 2022
MAURA: We’re starting this episode off a little differently this week. Usually we begin each episode with a dive into the Niels Bohr Library and Archives, but today I have the privilege of starting this episode in the Allegheny Observatory in Pittsburgh, Pennsylvania. We’re in a circular room with a domed ceiling made of wood, and there is a slit that lets some light in. In the center of the room is a 16-inch telescope, the Keeler Memorial Telescope. And instead of our usual tour guide, Allison, I’m with Lou Coban.
LOU COBAN: I am the manager of the University of Pittsburgh’s Allegheny Observatory.
MAURA: And can you tell us a little bit about where we are?
LOU COBAN: Okay. So we are inside the dome of the Keeler Memorial Reflecting Telescope. The space in here is actually kind of rather amusing, because we had a very old telescope in here that was very, very large at one time. Now we have a more modernized telescope that’s much, much shorter. So this is a very large telescope dome, and it’s a very small telescope inside the dome. And this is basically sort of a cover for the telescope so that we don’t get rain or anything like that on it, and it also shields it from the wind. The floor stays fixed in this particular telescope, but the dome does rotate. As a matter of fact, it’s kind of funny. A lot of times when we have students in here and we move the dome, since the walls are rotating, it will oftentimes make people — it makes people sick, so they start getting like some kind of vertigo thing going on or something like that, and you have to tell people to close their eyes if they feel sick.
MAURA: You’re right. That is funny. And you mentioned you sometimes have students here from the University of Pittsburgh. So the Allegheny Observatory’s telescopes are used for research.
LOU COBAN: Yeah. So we have a group here at the University of Pittsburgh called the STEPUP Group, which stands for Survey of Transiting Extrasolar Planets at the University of Pittsburgh. And that group uses this telescope to find transits, which are planetary transits across the front of the — across the face of their parent star. And so what it does is it causes a very slight light dip as the planet blocks out the light from the star. It’s very small. It’s about 1 percent, maybe about a tenth of a percent sometimes. But that’s actually quite visible.
MAURA: And the telescope, Keller, is named for a former director of the Allegheny Observatory.
LOU COBAN: Yeah. So Keller was the second director of the Allegheny Observatory, and I believe he started probably in about 1890 or so, and I think he left for the Lick Observatory in the late 1800s.
MAURA: And what were some of his major contributions to the observatory?
LOU COBAN: Actually, he made a really neat observation — set of observations with the 13-inch telescope at the old observatory. So this is an old 13-inch refracting telescope. And he used that telescope to figure out that the rings of Saturn are made up of small particles. Now back in those days, everybody thought you could get out of your aerial spaceship and walk around on the surface of the rings. They thought that they were solid. But what he did is he used spectroscopy to look across the ring plane, and he figured out that the inner edge of the ring is rotating much, much faster than the outer edge. So that means that the rings are basically rotating differentially, which means they’re following Kepler’s laws of planetary motion, which means that they’re made up of small particles.
MAURA: If they were solid, the outer rings would be moving more quickly than the inner rings, just like a disk on a stereo. But Lou, you do more than just manage the observatory. Sometimes you give tours of the observatory.
LOU COBAN: Yes. So we do tours on Thursday and Friday evenings of the building, and the tours are always free. And the reason why, as John Brashear said a long time ago, that the Allegheny Observatory shall remain forever free to the people. And so, to this day we are still able to offer free tours.
MAURA: And though I’m here on a Sunday, can I have one of these said tours?
LOU COBAN: Sure, for 50 bucks. [laughs]
MAURA: [laughs] Alright. So let’s continue our tour. We’re going to descend a kind of rickety staircase.
LOU COBAN: This is actually sort of a non-public area of the building. So if you come on one of the tours actually, [laughs] unfortunately you would not see this area of the building. Only students get to see this part of the place.
MAURA: And physics history podcasters.
LOU COBAN: Yes. [laughs] Exactly.
MAURA: So now we’re walking down this marble staircase into an atrium of sorts.
[5:00]
LOU COBAN: Actually, it’s called the Rotundra, or as one of the old janitors used to call it, the Rotundrum. I have no idea why.
MAURA: So inside is this beautiful glowing stained glass window.
LOU COBAN: Okay. So this figure that we see in the stained glass window is Urania, the Greek muse of astronomy. And there’s a lot of symbological elements inside the window. For instance, she’s holding in her hand sort of a round orb, which represents the celestial sphere. There’s also the Pleiades and the Hyades clusters above her hand that she has raised up into the sky. We have the lamp of knowledge also in the window, and the Acropolis in the background. And more interestingly, we have sort of a rainbow down at the bottom of the window. And that represents light split up into its component colors. And so one of the great things that they were doing at the turn of the century — and one of the great discoveries — was figuring out what stars were made up of by their spectrum. So the spectra that they were getting from the stars gives them an idea of what the stars were made of. And so that was sort of a big thing, and that’s what that rainbow is there for.
MAURA: This is normally where I’d say, “From the Niels Bohr Library and Archives at the American Institute of Physics, this is “Initial Conditions,” a physics history podcast. But we’re not in the Niels Bohr Library and Archives at the American Institute of Physics. So instead, we’ll say —
LOU COBAN: From the Allegheny Observatory in Pittsburgh, Pennsylvania, this is “Initial Conditions,” a physics history podcast.
MAURA: Nailed it. Every physics problem begins with a set of initial conditions that provide the context for physics to happen. Likewise, in “Initial Conditions,” we provide the context for which physical discoveries happen. I'm your host, Maura Shapiro, and Justin will join us in a little bit later in the episode. This week, the subject of our episode is looking at me right in the eye. Lou, there’s not exactly an elephant in the room, but there is a giant statue.
LOU COBAN: Yes. This is the statue of John Brashear, who was sort of the patron saint of the new Allegheny Observatory. So the original observatory was built in 1860, but the new facility, they broke ground on it in 1900. And John Brashear was the person who was very responsible for getting a lot of the funding together to create and construct the new Allegheny Observatory.
MAURA: And Brashear was important more than just creating the Allegheny Observatory. His lenses transformed our understanding of light. I have to admit, an episode of “Initial Conditions,” a physics history podcast, would not be the same without my intrepid co-host, Dr. Justin Shapiro.
JUSTIN: Good morning, Maura. I hear today you’ve got a story that’s very near and dear to your heart. And I also want to say it’s near and dear to my heart and where I grew up, because it takes place in Pittsburgh, Pennsylvania, an underrated gem: “the Paris of Appalachia.”
MAURA: As it’s known, “the Paris of Appalachia.” So basically since we’ve started this project, the podcast, I’ve been wanting to tell this story, because as you mentioned, it hits close to home, and I have been known to cry while telling this story. So I have my tissues. If I cry, just bear with me. But without further ado, let’s talk about the unexpected hero of light.
JUSTIN: What are our initial conditions for today, Maura?
MAURA: Our first initial condition is Maxwell’s equations and the advancement in understanding electricity and magnetism in the 19th century. Our second initial condition is industrialization in Pittsburgh, Pennsylvania, which turned it into the “steel city” that it’s thought of today. And our third initial condition is European dominance in astronomy and optics.
JUSTIN: Those all seem like pretty different initial conditions, and I’m excited to see how they all piece together.
MAURA: And I’m excited to talk about it. So let’s start with light. Light is super cool. I mean, we take it for granted, but it truly is the key to everything. Using light from distant stars, we can know the atmospheric makeup of planets that orbit that star. We can determine how fast the planet is traveling, and maybe even how close it is to its star. But light is cool for so many other reasons, and up until recently, with the detection of gravitational waves, everything we knew about space and the universe came as information coded into light and detected by telescopes. So today I’ll tell you a story about how our understanding of light changed
[10:00]
in the 19th century and the surprising role an observatory just across the river from Pittsburgh played in our understanding of light.
JUSTIN: And I’ll be interested to know how Pittsburgh — which was a very smoky city in the late 19th century — played a role in this story. And I understand that maybe you’re interested in the subject for another reason.
MAURA: Yeah. That’s true. The observatory in question is the Allegheny Observatory, where I did my undergraduate research. As a physics student, going to the Allegheny Observatory just felt super important. It’s perched on top of this hill in a large park, and it has this regal air of a temple of astronomy. The entrance is framed with Ionic columns, and resting on top of the building are three massive protruding domes that house the telescopes. And inside, there’s this glamorous and echoey hallway and a beautiful multistory library with images of great astronomers watching over you as you work. There’s a little atrium with a glowing stained glass window and a life-sized statue of John Brashear.
JUSTIN: That sounds like a really neat place to do research. But I’m still missing the link between the Allegheny Observatory and our understanding of light.
MAURA: Fair enough. So this episode just be a nostalgia trip for me, so cut me off if I get too sentimental and forget what we’re talking about. But let’s start with the very basic question that’s at the focal point — that’s an astronomy pun — of our episode: what is light?
JUSTIN: Wait, do you want me to answer?
MAURA: Yeah.
JUSTIN: Whew. Okay. Well, off the top of my head, there is this thing called the electromagnetic spectrum that encompasses all different sorts of different radiating energies, including radio waves with very low wave frequencies, microwaves with higher frequencies, X-rays with higher frequencies, and gamma rays with the highest. Each type of radiating energy has a different ability to penetrate different materials, and that’s why X-rays can go through skin but bounce off of bones. Light is the visible portion of the electromagnetic spectrum for humans. Different wavelengths of light, all of which are contained in rainbows, bounce off of different objects and are received by our eyes. Plants, for example, look green because chlorophyll reflects green light. Oh, and also, visible light travels at a constant speed in a vacuum, the “c” of “e=mc2.”
MAURA: Great. And for our purposes, light is just the entire electromagnetic spectrum. But we didn’t always know light was like this. For simplicity, let’s start with how Newton understood light. We’ll call this the “classical understanding of light.” And he thought — and many people agreed with him for hundreds of years after — that light was made up of little particles that shot straight from a light source into your eyeballs, traveling through a medium at an infinite speed, which would mean that it reaches its destination instantaneously. Now, other Islamic scholars had believed that light traveled at a finite speed, but either way, a medium was always implicit.
JUSTIN: And by “medium,” you don’t mean, like, halfway between big and small. It’s more like a medium as a substance, as something to pass through.
MAURA: Right. Exactly. And this light medium is a substance that we couldn’t see, but somehow it permeated everywhere light needed to be. And the name for this medium is “the luminiferous ether.” And I’ll say that as much as possible, because it’s just fun to say. Try it.
JUSTIN: “Luminiferous ether.” I also seem to remember that we talked about it back in Episode 5, and I have a sense you’re going to build on that.
MAURA: Yeah, and we’ll talk about that a little bit later. But moving forward in our history of the study of light, people realized that light was, in fact, traveling at a finite speed, and there was some effort in understanding how fast light travels. Spoiler alert: it’s pretty fast. So Galileo proposed an experiment that involved shining lanterns on distant hilltops, but it wasn’t super important to have an accurate measure of the speed of light. And that’s fine, because the technology needed to measure it just simply did not exist at the time of Galileo. But as time went on, physicists studying light started to realize that it wasn’t behaving as expected. It behaved like a particle sometimes, and other times, a wave. Thomas Young performed the famous “double-slit experiment” in 1801 that demonstrated the wave-like behavior of light.
JUSTIN: Thomas Young was an interesting person. He was also an Egyptologist and a linguist, and was perhaps the first person to translate the Rosetta Stone.
MAURA: Yeah. He was a man of many talents, and he wasn’t the only person interested in the form of light. Outside the double-slit experiment, light also behaved as a particle. Naturally, physicists were puzzled at light’s confusing behavior. Still, light as a wave made the luminiferous ether all the more logical, because waves are literally defined as a disturbance in a medium.
[15:00]
JUSTIN: Right. You mentioned a couple episodes back that the way scientists understood waves in the early 19th century is that they required a medium. So, saying light traveled in a vacuum made no sense. It would be like saying a boat could float without water.
MAURA: Exactly. The luminiferous ether just made sense. So we’ll go back to the ether in a little bit, because I want to keep chugging through our history of light. So also around this time, scientists are looking at the spectrum of light, which you can see when light shines through a prism. And different wavelengths of light separate. This is a field called “spectroscopy.”
JUSTIN: Scientists thought light was just the visible spectrum, because for most of the history of what we know about light, we hadn’t discovered non-visible light or didn’t realize it was part of the same phenomenon.
MAURA: Exactly. But by the second half of the 19th century, we come to understand that by studying the spectrum of light coming from a star, you could deduce the elements that make up that star. By combining the subject of astronomy and methods of spectroscopy, you get a new field: astrophysics. Where astronomy had been primarily concerned with finding and tracking the orbits of celestial objects, astrophysics focused on analyzing the light coming from those celestial objects to learn more about them. And that’s where the Allegheny Observatory first comes into play. Samuel P. Langley, the first true director of the Allegheny Observatory, was also one of the early adopters of astrophysics.
JUSTIN: You said “the first true director.” I assume that means it was around in some other form before Samuel Langley.
MAURA: Right. Flashback to 1850s Pittsburgh. It was very much an industrial town led by these wealthy industrialists who controlled the railroads and the steel mills. And they get together and they form the Allegheny Astronomy Club, and they decide to build a telescope, but not just any telescope. They wanted to buy the biggest telescope possible. So the Allegheny Astronomy Club procures this giant — at the time — telescope and builds an observatory. But the Civil War breaks out, and most of these industrialists’ attentions go elsewhere, and the club is no longer functional. Its expenses are too much to keep up with, and they sell it to the Western University of Pennsylvania, which is the precursor to the University of Pittsburgh, which is the school I went to. William Thaw was a railroad tycoon and also one of the founding members of the Allegheny Astronomy Club. He was still really invested in the observatory, because he wanted it to be more than just a hobby for rich people. He wanted it to be a research institution and open to the public for learning. Keep in mind, the Allegheny Observatory had one of the biggest telescopes in the world, and it was simply going to waste.
JUSTIN: So at this time, the Allegheny Observatory is part of the Western University of Pennsylvania, and they hired Samuel Langley to be the director.
MAURA: Right. Langley was born in 1834, and even as a kid, he excelled in math and astronomy. He became an assistant at Harvard’s observatory and started to make a name for himself as an astronomer. And he was a lifesaver for the observatory. He quickly found a scheme to fund the observatory by essentially setting up the precursor to standard time. A standardized time was crucial to railroad systems who relied on accurate timing to prevent railroad crashes. The railroad companies paid the Allegheny Observatory for access to their time. But Langley was more interested in studying the Sun, and specifically analyzing the light from the Sun, which as we just talked about, is astrophysics. And he was, in fact, one of the first writers of an astrophysics textbook, called The New Astronomy in 1887. And he was actually a super interesting person for a bunch of reasons. His solar observations were a huge deal and propelled him into the prestigious position of Secretary of the Smithsonian Institution. But he was also obsessed with flying machines and spent the latter part of his professional career researching how to make things fly. And he was fairly successful until a hugely embarrassing failure that occurred just a few days before the Wright brothers successfully flew their plane. He never recovered from the humiliation.
JUSTIN: Okay. That’s really interesting. I remember you once saying you first learned about Langley at the Air and Space Museum before even going to Pitt. But I am a little confused about how this all fits into the history of light and the luminiferous ether.
MAURA: Yeah, that’s fair enough. Really, the story I’ve been excited to tell is about one man: John Brashear. Actually, he was often known as “Uncle John.” His autobiography is called A Man Who Loved the Stars, and that’s really who he was — a guy who loved the stars and wanted everyone to be able to see them the way he could. He was born November 24, 1840, and grew up in a poor family outside of Pittsburgh. From an early age, his grandfather nurtured in him a love of astronomy. Together, they read books about the subject and would lay outside
[20:00]
at night, after it was too late for a kid to be out, just looking at the sky. And his love for the sky was cemented when his grandfather took him to see Saturn through a telescope. He immediately fell in love with the planet and its remarkable rings.
JUSTIN: I imagine Brashear seeing Saturn’s rings for the first time must have been a very profound experience.
MAURA: Yeah. It completely set him on his trajectory. But a love of the stars wasn’t the only thing he inherited from his grandfather. His grandfather was also a talented mechanic, and Brashear grew up to be one as well. He briefly pursued a career as a minister before moving to Pittsburgh to work in the steel mills. He was quickly promoted for his skill as an engineer despite having little more than an elementary education. He remained involved in religious affairs and was always very spiritual. Though he was always very involved in the church, we can assume a beautiful Sunday School teacher might have been an ulterior motive for his participation. Her name was Phoebe Stewart, and the two quickly fell in love. They married in secret and became partners in every sense of the word.
JUSTIN: What do you mean by that?
MAURA: They were best friends, and they shared everything. They complemented each other’s talents and were completely devoted to each other. He credits all of his accomplishments to her, and you’ll see her really influential role in his life soon enough.
JUSTIN: Okay. So Brashear is working in a steel mill and marries the love of his life. I’m really happy for him, but I’m still not sure what this has to do with light.
MAURA: Okay. Yeah. So they’re happily married, but money is pretty tight. But they both love the night sky, and Brashear wanted to look at it through a telescope like he did as a kid. But they couldn’t afford one. Because he was a mechanic, he decided he could just make one himself. He converted the shed in their backyard to his workshop, and every day after work, he and Phoebe would work together in the small shop until after midnight. He studied textbooks, even taught himself German, so he could read the best texts in the world. And after two years of exhausting work, the lens was polished and perfect. He holds it up to the light, and it slips through his fingers and shatters. I mean, it’s absolutely devastating. He put so much time and energy, and quite frankly, love and hope, into this one piece of glass, and it shattered. But Phoebe encouraged him to try again — do it so they could see the stars. So again, for two years, they skipped sleep, and they create another perfect 5-inch lens. He musters up the courage to write to Samuel Langley, the director of the Allegheny Observatory, who he knew was a highly respected astronomer, and asked if he could show him the lens. Langley happily invites him to visit the observatory. So Brashear wraps up this perfect piece of glass in a bandanna and makes his way up Observatory Hill. When he reaches the top, he’s super nervous, and he shows Langley the lens. Langley inspects it and recognized Brashear’s potential immediately. So he loans him optics books from his own collection and encourages Brashear to make a reflecting mirror for a reflecting telescope. Reflectors are much cheaper to make, because the glass doesn’t have to be perfect, so you can make a much bigger lens.
JUSTIN: I imagine the second time around, it goes better.
MAURA: Not exactly, but it still has a happy ending, I promise. Brashear sets up the telescope in his house with the 5-inch lens he already made, and the whole neighborhood travels to witness the sky as they’ve never seen it before. He sets about making his next lens, although at this point, people — and often children — are wandering about his shop to ask questions, which he always patiently answered. And this time, John Brashear procures enough glass for two 12-inch mirrors. Again, every day after work in the steel mill, he goes to his shop, which Phoebe arranges for him, and polishes this lens. Once it’s perfect and polished, he has to coat it in silver, which is how reflecting mirrors are made. And there are a bunch of different ways to coat a lens in silver, but he chose the most simple, popular method, and the lens cracked in two. He wrote, “The lens cracked in two, but my heart shattered.”
JUSTIN: I take it he tried again.
MAURA: Yeah. After a day of mourning, he recalled this telepathic voice from Phoebe, saying, “Crying over shattered glass won’t put it back together.” So he returns home from work to see her setting up the shop to do it all over again. This time, he consults multiple different textbooks. He writes to different authors, and he develops his own method of silvering a mirror, which is easier than other methods. He was successful, and he wrote to different magazines offering an explanation for his method. It ultimately became the standard method for coating a mirror in glass, and was called “the Brashear method.”
JUSTIN: So what did he do with this lens?
[25:00]
MAURA: Again, he walks up to the observatory to show Langley, but when he arrives, Langley was talking to someone else. He’s waved over and introduced to William Thaw, the observatory’s benefactor. Langley had talked Brashear up quite a bit to Thaw, and Thaw offered to sponsor Brashear’s lens-making.
JUSTIN: Brashear was still working full time in the steel mills, right? So lens-making was still very much a hobby for him.
MAURA: Yeah. And Brashear places an ad in the newspaper for his telescope-making services, but in typical humble Brashear fashion, the ad was more like an apology. He’s basically like, “I’m so sorry. You don’t have to buy a lens from me. Here is step-by-step how you can make a telescope by yourself. But if you really don’t feel like doing that, you can buy it from me.” And commissions came flying in. After effectively a nervous breakdown from overwork, Brashear finally quits his job in the steel mill because he's supported by his daughter’s husband and works full-time lens-making. Thaw supplements his income, because Brashear refused to charge even enough to cover materials for his telescopes. And Thaw also built a workshop for him next to the observatory. He made custom instruments to support Samuel Langley’s groundbreaking solar research, including a device that extended the known wavelengths of infrared radiation.
JUSTIN: So Brashear helped in discovering this invisible light that had previously been unknown.
MAURA: Right. And that’s not all, and I think this will be of particular interest to you, Justin. This device accurately measured atmospheric absorption of specific bands of infrared radiation, which inspired Svante Arrhenius’ climate change research.
JUSTIN: Yes. Arrhenius knew about infrared radiation, which has a slightly lower frequency than that of visible light. We can feel it as heat, and my cats can actually see some of it — shouts out to Bathmat and Ampersand — which, of course, was what interested Arrhenius. Not the cats, but the infrared radiation.
MAURA: And the atmosphere absorbing it. As Brashear’s lenses grew in popularity, he received a commission that catapulted him into international notice. But before I get into that, I want to say a little bit more about who he was. So Brashear is rising in fame and notoriety, and he’s friends now with all these wealthy Pittsburgh industrialists, but he always remained a man of the people. Even though he’s getting these big commissions, anyone could visit him in the workshop, and Phoebe would cook for them. He loved kids and constantly had a gaggle of them running around his workshop and mysteriously avoiding injury.
JUSTIN: Unlike Guy and Leslie Callendar, who we talked about along with Arrhenius back in Episode 2.
MAURA: Brashear just wanted astronomy to be as accessible as possible, so he lectured in churches and temples of all sects, and at schools, completely for free. He even visited the prison in Allegheny County to make sure the inmates could see the night sky through his telescopes. I say all this now because even after becoming one of the most important lens makers in the world, he remained like this, dedicated to making the stars, the night sky that he loved so much, accessible.
JUSTIN: Okay. I have to ask you again. You keep teasing that he’s going to do something big. What launches him into international renown?
MAURA: Yes. Okay. Are you sure you don’t want to keep talking about what a great person he was? Because I could. [laughs]
JUSTIN: Oh, I know you’ll continue to talk about great of a person he was, and I know you can. I remember when you were reading one of his biographies. Almost every hour, you would send Allison and me another anecdote via Slack about how wonderful and kind he was. It was very touching.
MAURA: Yeah. So let’s get down to the optics. After producing this beautiful lens for Lick Observatory, he’s commissioned to correct a diffraction grating for Henry Rowland, who was a very prominent physicist at Johns Hopkins University in Baltimore. Diffraction gratings were famously hard to make. Their lens is etched with parallel lines that split light into its different wavelengths, and they have to be super precise. Just for scale, Brashear and his team needed to polish a thousand parallel lines to an inch. So if you look at the top nub of your thumb, a thousand parallel lines had to fit into that. And this was a harrowing experience. This was really difficult for Brashear and his ever-growing team. But they made it, and they sent it to Rowland, who measured it against a grating he had, which was made by the best manufacturer in the world. But Rowland found that Brashear’s lens had a significant error.
JUSTIN: Brashear’s lens was no good?
MAURA: Not exactly. Fortunately, Langley was visiting Rowland and suggested that perhaps it wasn’t Brashear’s grating that was wrong, but the one he measured it against. And Langley was right. Brashear’s grating was effectively the best in the world. While other companies were making gratings of a couple centimeters, Brashear and his team were making gratings of a couple inches, with better precision.
JUSTIN: So now everybody who matters knows his name.
[30:00]
MAURA: Yeah. If you’re making a spectroscope, especially in the U.S. but also abroad, you wanted Brashear. Little old Brashear, who just loved the sky and wanted everyone to love it too, is now producing the best optics in the world, and also — much to the dismay of William Thaw, who sponsors him — Brashear is not really charging a lot for these lenses.
JUSTIN: And because of his work with spectrographs and lenses for Langley, physicists had a much clearer picture of the different wavelengths of light, which is significant in and of itself. But I’m really curious how the “luminiferous ether” plays into his work.
MAURA: Yeah. Well, this is the big culmination of the story, but by no means Brashear’s life work. To tell this story, we have to introduce another character, Albert Michelson. And I actually read a biography of him by one of his daughters which is super interesting, because he had two families, one of which he effectively forgot, and the other he was very close to. But I definitely don’t see him as the charming, lovable John Brashear. Still, he was similarly devoted to science and precision. Michelson was born in Poland in 1852, but his family fled to the United States because they were Jewish and were escaping the pogroms. They moved to a mining town out west.
JUSTIN: This was at the same time that thousands of people were migrating westward to search for gold and other precious metals.
MAURA: Michelson’s family never became wealthy, but they made a life for themselves, and at a young age, Michelson excelled at math and science. He was accepted to the Naval Academy after the governor of Nevada begged President Grant to extend his quota of recommended students, and the president was so impressed with Michelson that he did. He was always very detail-oriented and a perfectionist, which makes for a very good experimental physicist. After he graduated, he took a job as professor of physics and chemistry at the Naval Academy. And as he was performing a demonstration on measuring the speed of light, he realized he could improve it. Michelson’s scheme was to use a spinning mirror to reflect light, and using a tuning fork, he could measure the speed of the spinning mirror. He published his measurement for the new design in 1878. Guess how much more precise his measurement was than the previously accepted standard.
JUSTIN: Hm. With no previous knowledge, I’m going to guess 10 times more precise.
MAURA: 200 times more precise.
JUSTIN: Wow.
MAURA: That’s so much. So this was the most accurate measurement of all time, and it brought him tremendous fame. He’s not even 27 at this point.
JUSTIN: And the speed of light is important, because it crops up all over the place in physics, including Maxwell’s equations, which showed the relationship between light, electricity, and magnetism.
MAURA: Exactly. And I’m really glad you brought it up, because Maxwell formulated his equations assuming there was an ether, and everyone thought there was an ether, as we talked about earlier. It would have been absurd not to. But different experiments were showing seemingly contradictory results. There were some different ideas on what the luminiferous ether would look like. Scientists know how things travel in a medium, so they could determine what properties the ether would have to have. So for light, which travels very quickly, the medium would have to have a density similar to that of steel but with an elasticity of 3.6 billion times that of steel. Or, on the other end of the spectrum, it could have had an elasticity similar to that of steel, but its density would have to be 50,000 times less than the hydrogen atom.
JUSTIN: And the ether also has to be invisible, right? Because we can’t see it, yet it has to permeate all types of matter and have no gravitational resistance.
MAURA: Much of the early work on light assumed that the ether was stationary, and that Earth traveled through it. But there was also an idea that Earth dragged the luminiferous ether along with it, or that there was some type of ether wind. But for various reasons, neither of these were really favorites. So let’s assume that we have a stationary ether, and Earth is speeding through it at 67,000 miles per hour on its orbit around the Sun.
JUSTIN: That’s enough to get you a speeding ticket.
MAURA: For sure. But it also means that if you send light in two different perpendicular directions, one beam would be moving sideways in relation to the ether, and one will travel parallel to it. Michelson wanted to exploit this to measure the effect of the ether on the speed of light. Now he knew that he couldn’t measure the speed of light precisely enough to see this impact, but if you measure the speed of light against itself, you get an interference pattern. If light is traveling at the same speed, the peaks and valleys of the wave will line up distinctively. But if they’re shifted, it will also result in a very distinctive pattern. Michelson devises an experiment to split a beam of light in two: one going in the same direction as the Earth, and one perpendicular. He then recombines these rays of light
[35:00]
and looks at the interference pattern, and he sees that there’s no real effect. So just to explain a little bit more what he did: if you take two people who can swim the exact same speed and you put them in a river and have one swim horizontally out and back through the current, and the other swim with the current and then against the current, even if both swimmers go the same distance, the one who swims horizontally will arrive sooner. So he expected this result from light, which he assumed was swimming through the luminiferous ether. But he didn’t see it, so he blames the experimental design.
JUSTIN: And as a perfectionist, I imagine he keeps working on it.
MAURA: Yeah. At this point, it’s 1881. He’s in Cleveland working at the brand-new Case School of Applied Science. And this whole time, he’s also making better and better speed of light measurements. He teams up with Edward Morley, who is a chemistry professor at Western Reserve University, to improve his experiment. And they wanted to rule out all experimental flaws that are possible, so they bounce light back and forth multiple times to increase the supposed effect of the ether. They even mounted the device on mercury, so they could spin it smoothly to observe the effect of different orientations. They also worked in the basement at night to limit the effects of traffic or even someone walking. Their device was so sensitive that someone walking 50 meters away could impact the readings. And they conducted their experiment over the course of months to see if the position of Earth in its orbit impacted it. But most importantly, they recruited the best lens maker in the world.
JUSTIN: Oh, John Brashear.
MAURA: John Brashear! Yeah. Nothing but the best could be tolerated for this precision measurement. But still, nothing. There’s no luminiferous ether. They published their results in 1887, and the so-called Michelson-Morley Experiment is widely cited as the experiment that killed the ether. Michelson went on pursuing the ether and speed of light measurements. He even performed a measurement for the wavelength of light that is now used to define the official meter for the International Bureau of Weights and Measures using, again, Brashear’s precise optical instruments. Brashear’s lens for Michelson’s interferometer was actually called “the flattest surface on Earth.” Now many people, even Michelson, didn’t actually give up on the idea of the ether in 1887, but it definitely laid the foundation. Albert Einstein’s 1905 Theory of Special Relativity — which proclaimed the constant speed of light in a vacuum — was really what gave ether its final blow, in my mind. Accounts differ as to how much Einstein was inspired by Michelson’s work, but it’s clear that it set up special relativity.
JUSTIN: It sounds like a lot of anomalies are piling up, and maybe the old paradigm isn’t able to sufficiently resolve them. In any case, this was all made possible because of the Pittsburgh lens maker, John Brashear.
MAURA: Yes. All of this is made possible by Brashear. He received many awards, but the award that meant the most to him was his honorary doctorate of science from the University of Pittsburgh. And there’s this really beautiful scene in his biography when he achieves this honor. At the time, the University of Pittsburgh was still the Western University of Pennsylvania and was situated next to the observatory. And he marches in his new doctoral robes up the hill, just like he did many years before, when he first went to show Langley his humble 5-inch lens, wrapped in nothing but a bandanna. And now, even though he has very little formal education, he receives a doctorate degree. He felt so lucky that after 25 years of working in a steel mill, he now had the career of his dreams and wanted to give back. He ultimately served as temporary director of the observatory and temporary president of the university. He actually became well known for his work in education, separate from his work as a lens maker. His goal, of course, was to make education accessible for all. He was generous with scholarships and almost anyone who asked would receive funding, including the woman who became the first woman educated at the Western University of Pennsylvania. He was also very involved in different civic associations and was on the board for School of the Blind, where he told stories about the night sky, so the children there could still “see” the stars. His wife Phoebe eventually sustained a crippling ankle injury and could no longer walk, so he nursed her full time. When she passed away, it broke his heart.
JUSTIN: Sounds like he gave a lot to his community.
MAURA: Yeah. By almost 80, he was still sharp but was sometimes forgetful. And by this point,
[40:00]
most of his best friends had passed away, and he was sick from all of his travels, from which he never fully recovered. Sometimes, he would gaze at the night sky and forget the names of the stars that meant so much to him. He even sometimes forgot the name of Saturn, the planet that first captivated him. But he never forgot the name of Saturn’s ninth moon, Phoebe. He died April 8, 1920. His ashes were put in the same urn as Phoebe’s and placed in the crypt of the Allegheny Observatory, with an inscription that reads, “We have loved the stars too fondly to be fearful of the night,” which is the final line of a beautiful poem by Sarah Williams called “The Old Astronomer to His Pupil.”
JUSTIN: It’s really beautiful that he always found comfort in the stars, and in some sense, will always be among them, resting at the Allegheny Observatory.
MAURA: Brashear was inspired by the light that traveled millions of miles from distant stars. He called them “the lantern which illuminated my steps at night,” as if made just for him. Today we understand light as electromagnetic radiation, which extends from the smallest wavelength, gamma rays, to the longest, radio waves.
JUSTIN: Light acts as both a particle, called a photon, and a wave. But the wave isn’t like a sound wave or ripples in a pond. It’s more like a signature, some information that reveals its existence. We also know that light travels at a constant speed in a vacuum and that nothing can travel faster than the speed of light.
MAURA: Understanding light is even the basis of modern physics. It’s important for relativity and quantum mechanics.
JUSTIN: So Maura, why is it so important to measure the speed of light?
MAURA: It’s a fundamental constant that shows up all over physics, and other fundamental constants rely on us understanding and precisely measuring the speed of light.
JUSTIN: Everything electronic relies on signals of light. As you mentioned, we use light as a measuring stick for the meter.
MAURA: Light tells us how particles behave and also how distant stars are moving. It tells us about the earliest possible moments of our universe, from background radiation.
JUSTIN: So Maura, what’s your final thought on the story of Brashear and the history of our knowledge of light?
MAURA: The better we understand light, the better we understand the universe and ourselves. This humble lens maker, who simply loved the stars, is central to what we know about light because of his precise instruments, which he made with love. We’re going to end our story in a very unusual place, with our tour guide at the Allegheny Observatory, Lou Coban.
LOU COBAN: We are in the crypt of the Allegheny Observatory, which is the base of the pier of the Keeler Telescope, about four floors down. As a matter of fact, the Keeler Telescope is at the very top of the building, and the crypt entrance is in the sub-basement of the building, one floor below the main basement.
MAURA: And it’s sort of a narrow cylinder with a beautiful plaster ceiling, ordained with roses and grape leaves and a giant light.
LOU COBAN: Yeah. So John Brashear was the one who had all the work done inside this area to make it into a crypt.
MAURA: And John Brashear is also buried here in the crypt of the Allegheny Observatory.
LOU COBAN: Yes. So his cremains are buried with his wife, Phoebe. And so another interesting thing, I think, is a lot of people ask me — you know, because I’m rattling around the building all the time, in the middle of the night in the darkness — and people are like, “Well, aren’t you afraid because there are, like, five people buried? Aren’t you afraid that they’re going to haunt you or haunt the place?” And my answer to that is “no,” because they are the people who cared about the observatory, and they are not going to do anything harmful to anybody that’s inside the building. So if you do anything that makes a lot of noise or something, they might come and see what you’re doing, but they’re not harmful at all.
MAURA: Does it provide you any sort of comfort having them here?
LOU COBAN: Yes, it does, because I think they’re sort of a protective force.
MAURA: Special thanks to our tour guide, Lou Coban, for our tour today. To learn more about our discussion, find related photographs, blog posts, and transcripts for this episode, check out our website at aip.org/initialconditions or click the link in the episode description. “Initial Conditions” is generously sponsored by the Alfred P. Sloan Foundation. The episode was created, researched, and written by Maura Shapiro and Justin Shapiro. Allison Rein is our executive producer, with audio production and editing by Carrie Thompson. Special thanks to the wonderful staff of the Niels Bohr Library and Archives and the Center for History of Physics for supporting us in all our research needs. I’m Maura Shapiro, and you’ve been listening to “Initial Conditions” — from the Allegheny Observatory in Pittsburgh, Pennsylvania.
[45:26]
Avery, Gaul Harriet Lester, and Ruby Eiseman. John Alfred Brashear, Scientist and Humanitarian, 1840-1920, by Harriet A. Gaul and Ruby Eiseman. Philadelphia, PA: University of Pennsylvania Press, 1940.
This account of John Brashear includes quotes from his friends and tells a moving story of his life. From this book, it is clear how much the community cherished him.
Brashear, John A. A Man Who Loved the Stars: The Autobiography of John A. Brashear. Pittsburgh, PA: University of Pittsburgh Press, 1988.
John Brashear wrote this autobiography which brought me to tears numerous times. From his own account his love for astronomy and for his wife, Phoebe, shines.
Livingston, Dorothy Michelson. The Master of Light: A Biography of Albert A. Michelson. New York, NY: Scribner, 1973.
This is a very interesting profile of Albert Michelson who dedicated his life to measuring the speed of light. It was written by his daughter from his second marriage and her account of her father is not unbiased but provides an unique perspective into his personal life.
“Spectroscopy and the Birth of Astrophysics.” Tools of Cosmology. American Institute of Physics. Date Accessed August 31, 2022. https://history.aip.org/exhibits/cosmology/tools/tools-spectroscopy.htm
Brashear’s team made lenses for Henry Rowland’s spectrographs. This virtual exhibit from AIP’s Center for History of Physics does a great job of explaining the science and history of spectrographs and how they ushered in a new age of astronomy.
“The Speed of Light.” In Our Time. BBC 4 Radio, November 30, 2006. https://www.bbc.co.uk/programmes/p0038x9h
Understanding the race to measure the speed of light is really an important backdrop of this episode. This podcast also does a great job explaining the physics of light and features Dame Jocelyn Bell Burnell.
Spence, John C H. Lightspeed: The Ghostly Aether and the Race to Measure the Speed of Light. New York, NY: Oxford University Press, 2020.
This book contextualizes the episode well and the history of the study of light.
Undaunted: The Forgotten Giants of the Allegheny Observatory, 2012. https://www.amazon.com/Undaunted-Forgotten-Giants-Allegheny-Observatory/dp/B01LBTO8LU.
This film does a great job telling the story of the Allegheny Observatory, which we do not spend as much time as I would like in the episode. Of course I am partial, given I conducted my undergraduate research from the Allegheny Observatory, but this short movie always brings me to tears. From the observatory’s involvement in creating a standard time, to measuring infrared radiation from the sun and its absorption in the atmosphere, to early spectroscopy and determining the makeup of Saturn’s rings. And of course, John Brashear’s role in the Allegheny Observatory: making lenses, giving lectures, serving as director, and raising money for a new observatory.
Williams, Sarah. The Old Astronomer to His Pupil. naic.edu/~gibson/poems/swilliams1.html
The final line of this poem is inscribed on the epitaph of John and Phoebe Brashears’ ashes which are buried in the crypt of the Allegheny Observatory. This is a beautiful poem and I hope everyone enjoys it.
Special thanks to our guest, Lou Coban. Kerry Thomspon of Thompson House Productions produced this show. Allison Rein is executive producer. Initial Conditions: A Physics History Podcast is generously sponsored by the Alfred P. Sloan Foundation.
Sources and Collections Used
Brashear, John A. John A. Brashear; the autobiography of a man who loved the stars, edited by W. Lucien Scaife. New York: The American society of mechanical engineers, 1924.
Gaul, Harriet A., and Ruby Eiseman. John Alfred Brashear, scientist and humanitarian, 1840-1920. Philadelphia : University of Pennsylvania press, 1940.
Livingston, Dorothy Michelson. The master of light; a biography of Albert A. Michelson. New York: Scribner, 1973.
Michelson, Albert A., and Edward W. Morley. “On the Relative Motion of the Earth and the Luminiferous Aether.” American Journal of Science 34, no. 203 (1887): 333-345.
“Spectroscopy and the Birth of Astrophysics.” Tools of Cosmology. American Institute of Physics. Date Accessed August 31, 2022. https://history.aip.org/exhibits/cosmology/tools/tools-spectroscopy.htm